Main Page
Networked geothermal
Networked geothermal is a system of networked ground source heat pumps that delivers heating and cooling to connected buildings. Networked geothermal systems have been used for decades on college campuses and are currently being installed by gas utilities and others across the country as a safe and affordable way to deliver heating and cooling without emissions.
Networked Geothermal System Components
Ambient Temperature Loop
A single horizontal closed loop of pipe is installed in the street below the frostline, where the ambient temperature is maintained within a range of 40-90°F depending on local climate. The pipe, which lies in the right of way and connects all the buildings on the system, is filled with water or a mixture of water and glycol. The water is the heat exchange medium and carries thermal energy as it circulates through the loop.
Materials: HDPE Pipe
Thermal Source(s), Sink(s) and Storage
The temperature of the ambient temperature is maintained partly through vertical or deviated boreholes several hundred feet deep into the bedrock, allowing the water to pick up the stable ground temperature of the earth via geothermal heat exchange. U-shaped HDPE pipes are installed in approximately 6" holes, grouted, and connected to the horizontal loop. Boreholes can be located in the right of way of the street or adjacent spaces, such as parking lots.
The boreholes serve two main functions:
- They give the system access to additional thermal energy, beyond what the horizontal loop can provide.
- They allow the transfer of excess heat from the horizontal loop into the bedrock, which acts like a big thermal battery. Excess heat shed from buildings in hot weather is not wasted, but rather stored in the bedrock for use in the winter. (A percentage of that energy dissipates, but much of it is available for the system to access weeks or even months later.) This thermal storage is one reason networked geothermal systems are so efficient.
A backup heater and cooler (heat pump) can be attached to the system or the buildings to ensure optimal performance in the case of an unusual heating or cooling event.
Materials: HDPE Pipe, grout, distributed fiber optic cable (DFOS)
Opportunistic Thermal Resources
Additional thermal sources and sinks (such as data centers, supermarkets, office buildings, or municipal sewage lines) can be used as needed or available. In dense urban areas the system connects buildings with different heating needs, so energy is not wasted, but is exchanged or stored in the ground until it is needed.
Temperature Delivery
A circulating pump run by electricity moves the water through this closed-loop system, maintaining a temperature between approximately 40˚–90˚ Fahrenheit, the range in which ground-source heat pumps operate most efficiently. Water is delivered to buildings, in which a ground source heat pump pulls heat inside or rejects heat back into the loop to raise or lower the indoor temperature, and distributes temperature through its normal distribution system (i.e., radiators, vents, etc.).
Ground-source or Water-source Heat Pump
A ground-source heat pump in each building is plumbed to the horizontal loop and either extracts heat in cold weather or transfers excess heat to the loop in hot weather.
What is a heat pump?
The first heat pump was created in 1856 to dry salt from salt marshes. The first ground-source heat pump was created in the 1940s. The oil crisis of the 1970s increased interest in heat pump technology, and significant improvements have been made to the design over the last 50 years. In recent years, the desire to move away from fossil fuels, along with advances in refrigerants, have led to much greater use of heat pumps, especially in Nordic countries. In Sweden, one in five homes use ground-source heat pumps.
Materials
- [Video] 2m Introduction to Networked Geothermal
- [PDF] Definition of Networked Geothermal
- [PDF] Networked Geothermal FAQs
- Networked Geothermal Site & Design Considerations
- Networked Geothermal Decision Timeline for Municipalities
Networked Geothermal System Design
In temperate climates, the design temperature ranges from approximately 40-90°F, though different system designs have different ranges. The temperature of the water can also be regulated by connecting to local thermal sources and sinks—eg. a body of water, municipal sewage lines, waste water facilities—to stay between 40-90°F, the temperature range where heat pumps work most efficiently. A supplemental backup heater, chiller or air source heat pumps on the shared loop of water can help maintain the temperature within the desired temperature range during unusual heating or cooling events, if needed.
Systems should be designed not for the maximum heating and cooling need of each building, but for the combined needs, known as loads.An optimal system design balances the loads, so that while some buildings are drawing heat, others are shedding heat. This “load canceling” allows systems to be designed at approximately 80% of peak load, which means lower installation and operating costs.
Sharing and storing energy
The system works most efficiently when it includes buildings that use heating and cooling in different ways. Even in a temperate climate, an office building will tend to use cooling throughout the year because of all the warm computers inside of it. Thus even when the temperature is cold outside, that office building will be “rejecting” heat, returning the water into the system hotter than it was before. Homes down the street can then use that heat. The shared loop essentially transfers thermal energy from one building to another. This “synchronous load cancellation” results in a more efficient overall system by reusing thermal energy shed by other buildings that would be wasted otherwise.
If the other buildings on the system’s shared loop do not require that thermal energy, excess heat can be stored in the bedrock through the boreholes until it is needed. This storage results in “asynchronous” load cancellation, increasing the efficiency of the system further and over longer periods of time.
Interconnection and sizing
Networked geothermal systems are designed to interconnect to each other. Over time, a system can expand from serving a few buildings to serving an entire municipality or territory.
It is highly unlikely that a large group of mixed-energy-use buildings (i.e. office buildings, ice rinks, homes, supermarkets, and schools) will need the maximum amount of heating or cooling at the same time. Networked geothermal systems are thus sized for the likely peak heating and cooling load of the connected buildings, rather than for the sum of all the maximum heating or cooling loads of the individual buildings. This is possible due to the interconnectedness of the system, and the inherent energy sharing and load canceling.
The larger the system and the more diverse the energy needs of the connected buildings, the more efficient the system will be and the easier it will be to balance the thermal loads.
Feasibility & Selecting a site
The ideal site would supply energy to more than one building, with the total heating and cooling use of around 300 tons (this is the equivalent thermal energy use of between 75 to 100 homes).
Other good items to look for include:
- The ability to expand the system in the future
- Sources of thermal energy such as surface water, solar heating or waste water
- How many owners would beed to be consulted
- Major technical challenges or expensive retrofits such as steam heat
Feasibility study: Massachusetts Feasibility Study by Buro Happold Engineering
Engineering firms: List of designers and installers
Technical Writing Tools
- Template networked geothermal request for proposals (RFP)
- RFP evaluation score sheet
- Sample scoping study
Similar Definitions
Networked geothermal synonyms
Networked ground-source heat pumps, geothermal micro districts, GeoMicroDistricts, geothermal networks, community-style heat pump systems, community heat pumps
Similar technology
Thermal Networks/Thermal Energy Networks (TENs): A shared loop of water with or without boreholes attached. Water temperature is maintained through energy load cancellation (some buildings using heating at the same time others are using cooling) and through backup heating and cooling "assets" attached to the shared loop. These networks can include combustion heating systems (such as a gas boiler attached to the shared loop) or not. Thermal energy networks can also include boreholes and can have no combustion. Networked geothermal and Clean Thermal Networks are a subset of Thermal networks and Thermal Energy Networks.
Clean Thermal Networks (CTENs): A thermal network without any combustion. Networked geothermal without any supplemental backup boiler is one example.
5th generation district heating and cooling systems (5GDHC) or 5th Generation district energy: Systems are decentralized, bi-directional, close to ground temperature networks that use direct exchange of warm and cold return flows and thermal storage to balance thermal demand as much as possible. 5th gen includes all of the above but is not synonymous because although the bidirectional pipes allow for buildings with different needs to exchange thermal energy so that energy losses are reduced and efficiency enhanced, they sometimes use 2-pipe or 4-pipe distribution which achieves lower efficiencies than a single-pipe system.
What it's not (see image)
Geothermal Electric Power: Electric generation plant from boreholes that are deep enough (generally more than a mile deep) to access temperatures high enough to create steam or hot water to spin turbines to generate electricity.
Geothermal District Energy: A central heating plant uses steam or hot water from deep geothermal boreholes. The distribution pipes must be insulated to reduce temperature losses with distance and the system cannot grow beyond the size of the central plant's capacity. Additionally this system does not provide cooling.
Geothermal Building: A single building with a heat pump in the building which takes temperature (both heating and cooling) from water moved through shallow boreholes.
Summary of Outcomes of Networked Geothermal
Safety
Networked geothermal is safer than gas, since it uses water (non-explosive!) to deliver heating and cooling,
Efficiency
Ground source heat pumps in general are one of the most efficient heating and cooling systems known. By interconnecting ground source heat pumps, networked geothermal improves on that efficiency. For instance, an ice rink connected to the system will demand a lot of cooling from the system even during the winter, returning the water into the loop hotter. That heat can then be used by homes down the street. Additionally heat can be stored in the bedrock until it's needed. Together, this synchronous and asynchronous load cancellation makes networked geothermal the most efficient thermal energy system known.
Affordable Heat, Efficient Grid: Using Thermal Energy to Save Time, Money, and Energy (BDC Blog, September 2024)
Indoor air quality
Combustion in a building is associated with lower indoor air quality, which is correlated with asthma and heart disease. Networked geothermal does not use any onsite combustion, so it improves the indoor air quality.
Cooling
Networked geothermal delivers cooling in addition to heating. In a warmer world, access to cooling can be critical. Heat waves are already more deadly than any other severe weather event.
Emissions
Networked geothermal uses only electricity. The emissions therefore come from the local fuel mix used to create that electricity. In Massachusetts, any building connected to networked geothermal would reduce its emissions 60% in comparison to being on gas heat. As the electric grid sources more of its energy from renewables, these emissions will continue to drop.
Reduced water use
Commercial buildings generally use cooling towers for air conditioning. Cooling towers evaporate water to provide air conditioning. They typically use 20 to 50% of the building's water. Networked geothermal provides cooling without evaporation.
Reduced customer energy bills
Applied Economics Clinic predicts that, in Massachusetts, residential heating bills with networked geothermal system are likely to be significantly lower than gas heating bills. This prediction is based on gas utilities installing the system and amortizing the cost of the system across all customers as they do with the gas infrastructure.
Local energy independence & reduced price volatility
Gas is generally shipped in from several states away and the fuel price can be volatile. The temperature for networked geothermal is just beneath your feet and thus is less likely to be subject to national market swings.
Reliability
With the gas system, natural gas has to travel hundreds or thousands of miles from wellhead to end use. This distance can result in single point failures, like in January, 2019 when a gas pipe malfunction in Ohio cut off the gas supply for Newport, Rhode Island during a historic cold stretch. Since the source for networked geothermal’s energy is the local ground, as long as you have access to electricity, you will have access to that heating and cooling. If the system has batteries to power it in case of an electrical outage, a networked geothermal system can serve as an island of resilience for a neighborhood.
Reduced impact on the electric grid
Buildings are moving to electricity for more of their needs, intensifying the strain on the electric grid, especially during the winter. The more efficient the method of heating used, the less the strain on the electric grid. Networked geothermal will greatly reduce this energy peak since it is the most efficient method known.
Thermal storage
Networked geothermal boreholes can store heat in the bedrock to be used even months later.
Projects Installed in the U.S. and Canada
Networked geothermal, or networked ground source heat pumps, has been used for decades on campuses to heat and cool multiple buildings. From Texas to Toronto, networked geothermal systems have been shown to reduce energy use and cut cost and emissions in a variety of climate zones.
College & university campuses
- Colorado Mesa University (Grand Junction, Colorado)
- Xcel Energy case study of Colorado Mesa University install
- Weber State University (Ogden, Utah)
- Skidmore College (Saratoga Springs, NY)
Commercial developments
- Green Streetscapes (West Union, Iowa)
- Springwater Mattamy Homes (Markham, Ontario)
- Whisper Valley (Austin, Texas)
Ongoing installations
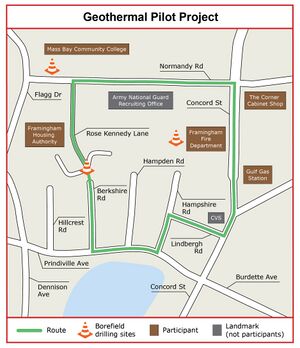
Eversource Gas
In the fall of 2022, Eversource Gas broke ground on the first gas utility-installed networked geothermal system in the nation. The system will provide heating and cooling to 37 buildings–32 residential and five commercial–for a total of 140 customers. Test well borehole drilling and thermal capacity testing has already been completed.
The utility, along with the City of Framingham and HEET have been selected as one of 11 recipients of the Department of Energy’s Community Geothermal funding initiative. The award of $715,000 will go towards planning the expansion of the first Framingham geothermal network, adding an additional loop.
Construction timeline and updates are available on Eversource's website.
National Grid Gas
National Grid Gas has selected Lowell, Massachusetts as the site for its first networked geothermal demonstration installation. The utility has a test borehole in the ground as of May 2023. See National Grid's video for more information.
Summary of Ownership models
The system can be owned by municipalities, developers, utilities, or others. Thermal networks should be deployed everywhere and anywhere, owned by anyone, however, most of this wiki focuses on the gas utility ownership model, because gas utilities transitioning from gas to geo will leverage already existing utility companies’ expertise, workforce and business model. Instead of networked geothermal competing with existing gas utilities, it provides them an avenue to use their workers and experience to aid the clean energy transition necessary in order to cut emissions fast, moving away from fossil fuels.
Equity
The utility of a utility is to pay the upfront cost of shared expensive long-lived infrastructure, maintain and operate it, and socialize the cost across all customers over decades. Utility companies already have the right of way in the street and expertise with pumping thermal energy underground. They also have the financing expertise to spread the cost of the system across all of us customers and over decades.
The utility ownership model provides clean, affordable, safer energy to everyone on the street, not just those who have disposable income or good financing (as happens too often with solar). Additionally, it reduces stranded assets from increased gas infrastructure investment. In order to maintain safe operation of the current gas system billions of dollars are required to repair leak-prone aging infrastructure, and since this cost is spread over all gas customers (rate-base), customers would be paying for assets in the ground for many decades. These assets or infrastructure will have to be retired before they are done being paid off due to the nation’s aggressive climate targets.
Having utilities spend the customer money on non-emitting infrastructure that can meet any decarbonization mandate and make the area a little more energy independent, might be a better way to spend that money than gas infrastructure.
However much of it is also applicable to other ownership models as well.
Policy changes needed for widespread adoption
Recommended reading Sightline Institute policy brief: Accelerating an equitable transition off gas
-
To allow and incentivize networked geothermal, legislative and regulatory policy changes will be needed. Specific changes needed will vary based on the policies of each state and what the aligned stakeholders want. Below is a list of suggested policy areas for legislators and regulators to address in order to allow gas utilities to install and operate networked geothermal systems and to allow the technology to scale. These changes are likely to go through several stages as networked geothermal becomes increasingly understood, trusted and normalized.
Stage 1: Pilot
Fund a feasibility or scoping study through ratepayers or the state. A feasibility study examines the overall potential for a networked geothermal project in a particular area, given factors such as climate, geology and energy use intensity. A scoping study searches for sites that would be financially attractive.
Fund demonstration installations through ratepayers or the state or other funding. The installations would benefit the ratepayers through exploring a model that can meet the state's emission mandates while reducing the likelihood of the gas utility assets becoming stranded as the customers defect to air source heat pumps.
Examples of rate cases:
- Eversource Gas Rate Case
- Approval Order (DPU 19-120)
- HEET's Brief
- National Grid
Ensure maximum learning and trust from the installations. The point of any installation or study is increased understanding and trust. Thus it is critical to ensure the results of installations are studied by independent experts trusted by all sides and to share data with maximum transparency. In Massachusetts, for example, an independent research team funded by the Massachusetts Clean Energy Center will study the first installations and create a public databank, including best practices.
Experiment with customer billing and metering. Networked geothermal can be billed via gallons of water entering a unit, or through BTUs (British Thermal Units), or through a monthly customer service charge based on expected peak load.
Stage 2: Permit Scaling
Define networked geothermal as non-combusting and utility-scaled.
Allow gas utilities to install and fund networked geothermal. Most gas utilities are currently only legally allowed to sell gas. This must be changed for utilities to install networked geothermal widely.
Allow gas infrastructure funding to be redirected towards networked geothermal infrastructure along street segments where the gas infrastructure would otherwise have to be upgraded.
Merge the gas/networked geothermal rate base. As customers choose to electrify buildings and move off the gas system, a reduced gas customer base will be left shouldering the costs of the same sized system with the same operations and maintenance costs. mer heating bills. Rising gas customer bills can make customers leave the gas system even faster, resulting in a vicious cycle that will result in stranded assets. Merging the gas and geothermal rate base allows the gas system (and customers and workers) to transition from one system to another all within the same rate base, avoiding the reduction of customers that leads to rising energy bills and stranded assets.
Allow for thermal service and infrastructure to be installed, for thermal service to be equivalent to gas, for the "obligation to serve” new customers to be met with thermal service, rather than with gas. Ensure that the gas utility franchise in each municipality is modified to include the ability to install thermal infrastructure.
Disincentivize investment in new gas infrastructure at a speed that meets the local emission mandates and reduces the potential for customer price shocks.
Use performance-based incentives to give gas utilities more profit for non-combustion infrastructure, such as networked geothermal, and less profit for combustion infrastructure.
Examine regulations around borehole drilling. In some states, boreholes are considered water wells and therefore are regulated under the jurisdiction of the local board of health. If a networked geothermal system contains just water with no glycol or other harmful chemical in it, and any slurry and environmental impact from the drilling is properly controlled, health and environmental impacts would be minimal or nonexistent.
Examine regional driller certifications to ensure they allow for the workforce to scale and work in the street. If the certification can be as similar as possible to nearby states, drillers will have a regional zone within which to work, attracting more of them.
Stage 3: Equitable Transition
Consider changing electric rates for heat pump owners to reward customers for helping the state meet its emission reductions. Reducing electric rates for heat pumps will also help low-income customers transition to electricity and enjoy improved indoor air quality in their homes without being financially penalized. Ground source heat pump customers in particular should be rewarded for the benefits to the electric grid of reducing electric peaks in comparison to other methods for electric heat, while increasing the overall amount of electricity being sold ("load factor").
Examine the potential for electric utilities to pay for a portion of the building retrofits based on those benefits to the electric grid.
Use a special purpose vehicle through section 1706 of the Inflation Reduction Act to get a large loan ($500 million or more) from the Department of Energy’s Loan Program Office. Loans from the LPO are backed by the federal government and have significantly lower interest rates than what most utilities can get. As a requirement of the loan, cost savings from the lower interest rate are spent on customer retrofits and/or worker retraining. In Massachusetts, using 2021 data, the cost savings were roughly equivalent to $16,000 per unit (home or business).
Allow current gas workers to transition in their jobs, keeping their salary and benefits. Gas and water pipes (used for the shared horizontal loop in a networked geothermal system) are made of the same material as gas pipes. Gas workers are already certified to operate and maintain this part of the system and can easily be retrained to operate other parts.
Create a tactical thermal transition tool mapping future investments in gas infrastructure, electric grid constraints, building stock, geology, energy use intensity, and any other data layers necessary to find the fastest and least expensive method to transition.
Experiment with methods of customer acquisition. Publish all the street segments where gas infrastructure will have to be upgraded (whether replacing vintage pipe or upgrading for gas constraints). Try out having gas utilities or independent vendors contact the customers to explain options.
Stage the customer retrofits using multi-modal heat pumps. There are now heat pumps that can pull temperature off of either the air or the ground, depending on what equipment they are connected to. This means that as the combustion HVAC in the buildings reach their end of life, they can be transitioned to heat pumps and connected to an outside air source compressor. When networked geothermal reaches that street, the heat pumps in the building can easily be connected to the system. The outdoor air source compressor can then be moved to wherever it is needed by another customer.
Allow for neighborhood electrification with avoided costs. Where networked geothermal is not financially viable, allow the gas utility to move the customers to electric heat, using the avoided costs to replace all gas appliances with electric or propane appliances.
Relevant legislation
Summary of Legislation Passed Regarding Thermal Energy Networks
A Legislative Heatwave (Updated June 2024) - Building Decarbonization Coalition
Colorado - Concerning the implementation of measures to advance thermal energy service, HB23-1252, (passed in 2023)
Illinois - Thermal Energy Network and Jobs Act, HB2875, (submitted in 2023)
Maryland - Geothermal Heating & Cooling Systems, HB 1007, (passed)
Massachusetts -
- Future of Clean Heat, H.3202, S.2105, (submitted)
- An Act Driving Clean Energy and Offshore Wind, H5060, (passed in 2022): Section 57 of the bill authorizes pipeline replacement funds to be used for non-emitting renewable energy infrastructure and incentivizes gas companies to make long-term repairs rather than expensive replacement of old pipes.
- An Act Creating a Next Generation Roadmap for Massachusetts Climate Policy (passed in 2021): Section 22 of the bill permits gas utilities to do pilot installations of utility-scale renewable thermal energy and allows utilities to bill customers for thermal energy.
- An Act For Utility Transition to Using Renewable Energy (passed in 2020) outlines a safe, just transition to move Massachusetts utilities off gas and shift customers to clean energy in alignment with state mandated greenhouse gas reduction targets and requires the Department of Public Utilities to consider equitable access to energy efficiency and renewable energy.
Minnesota - Natural Gas Innovation Act, 216B.2427, (passed)
New York - Utility Thermal Energy Network and Jobs Act,, S9422, (passed in 2022)
Vermont -
- An Act Relating to Affordable Community Energy Solutions H.56, (submitted in 2023)
- An Act Relating to Thermal Energy Networks, H.242, (submitted in 2023)
Washington -
- An Act relating to promoting the establishment of thermal energy networks, HB. 2131, (passed in 2024)
- Supporting Washington's clean energy economy and transitioning to a clean, affordable, and reliable energy future, HB. 1589, (passed in 2024)
Research
Learning From the Ground Up (LeGUp)
In order to scale networked geothermal and ensure financial viability, we need more research. HEET, a Boston-based climate solutions incubator, formed the Learning From the Ground Up (LeGUp) research team to collect data from the first utility-led installations of networked geothermal in Massachusetts. The team will develop technical, scientific, equity, and economic reports to inform the scaling of this technology and create a pathway to decarbonize natural gas heating throughout the country.
Massachusetts Clean Energy Center awarded HEET a $5 million grant for the multi-year research effort. MassCEC is engaged with HEET to monitor the impact of the research and identify additional opportunities to promote Massachusetts’ climate goals.
The research collaborative:
The LeGUp team is made up of top scientists and experts from across the country, including researchers from:
- National Renewable Energy Laboratory
- Lawrence Berkeley National Laboratory
- Massachusetts Institute of Technology
- Salem State University
- Buro Happold Engineering
- Boston University
Research Areas and Outputs
The LeGUp research team will:
- Provide feedback on the design and deployment of networked geothermal projects
- Develop models of system installation and operation
- Identify cost drivers and barriers to adoption
- Recommend opportunities to improve efficiency, equity, and integration of networked geothermal with other services
- Develop data-driven recommendations for scaling networked geothermal throughout the state
- Start a networked geothermal public data bank, a collection of normalized, shareable data from each installation to facilitate learning and replicability.
- Launch a feasibility study program to help interested communities explore networked geothermal as an option for decarbonizing
- Document and disseminate findings through reports, media (academic, industry, and general), stakeholder engagement, and educational materials.
- Evaluate the impact of transitioning from natural gas to networked geothermal on human health and wellbeing, methane emissions and the environment.
- Examine impacts on low income and environmental justice communities and provide recommendations on how to best distribute benefits.
Research will be carefully designed to supplement National Grid and Eversource’s own measurement and verification processes of their projects.
Research Papers
- Grid Cost and Total Emissions Reductions Through Mass Deployment of Geothermal Heat Pumps for Building Heating and Cooling Electrification in the United States, Oak Ridge National Laboratory (Department of Energy), 2023. Xiaobing Liu, Jonathan Ho, Jeff Winick, Sean Porse, Jamie Lian, Xiaofei Wang, et al.
- Inefficient Building Electrification Will Require Massive Buildout of Renewable Energy and Seasonal Energy Storage, Scientific Reports, 2022. Jonathan J. Buonocore, Parichehr Salimifard, Zeyneb Magavi & Joseph G. Allen
- 5th generation district heating and cooling systems: A review of existing cases in Europe, Renewable and Sustainable Energy Reviews, 2019. Simone Buffa, Marco Cozzini, Matteo D’antoni, Marco Baratieri and Roberto Fedrizzi
- Bidirectional low temperature district energy systems with agent-based control: Performance comparison and operation optimization, Applied Energy, 2018. Felix Bünning, Michael Wetter, Marcus Fuchs and Dirk Müller
- Design considerations for borehole thermal energy storage (BTES): A review with emphasis on convective heat transfer, Geofluids, 2019. Helge Skarphagen, David Banks, Bjørn S. Frengstad, and Harald Gether
- Influence of building heat distribution temperatures on the energy performance and sizing of 5th generation district heating and cooling networks; Energy, 2023. Maccarini et al.
Conference and seminar content
In-person or limited-attendance conferences and seminars provide a wealth of information and resources. This section is dedicated to debriefing and sharing content from such events, where expertise, advice, or points of view on networked geothermal might be shared, along with other related resources.
Networked Geothermal track at NY-GEO Conference 2023
- Mapping the Journey to a Thermal Market (slide deck, video)
- Measuring Success: The Data We Need" (slide deck, video)
- The Policy and the People We Need to Clear the Path Ahead" (slide deck, video)
- Utility of a Utility" (slide deck, video)
- Scaling Efficiently: How We Will Build a Thermal Grid Together" (slide deck, video
Resources
Networked Geothermal 101
- [Video] 2m Introduction to Networked Geothermal
- [PDF] Definition of Networked Geothermal
- [PDF] Networked Geothermal FAQs
- Networked Geothermal Site & Design Considerations
- Networked Geothermal Toolkit
Ground source heat pumps 101
- Clean Energy 101 on Geothermal Heat Pumps (Rocky Mountain Institute)
- Ground source heat pumps environmental impact (Dandelion Energy)
- Air source versus ground source heat pumps
- Geothermal heat pump manual
Networked Geothermal 102
- Thermal Energy Networks info sheet (Egg Geo, LLC)
- District heating system ownership guide
- Business models for district energy: A continuum from public to private
Technical Expertise
Technical Writing Tools
- Template networked geothermal request for proposals (RFP)
- RFP evaluation score sheet
- Sample scoping study
- Site selection checklist
Relevant Associations
To get connected to organizations listed below, email wiki@heet.org.
International Ground Source Heat Pump Association (IGSHPA) -An international organization works to advance ground source heat pump (GSHP) technology on local, state, national, and international levels.
GeoExchange Organization (GEO) - The voice of the geothermal heat pump industry in the US.
NY-GEO (New York Geothermal Energy Organization) - A nonprofit dedicated to promoting geothermal heating and cooling.
Utility Networked Geothermal Collaborative (UNGC) - A national coalition of gas utilities learning and working actively toward networked geothermal.
Geothermal Rising - Formed in 1972, Geothermal Rising is the oldest geothermal association on Earth, serving as the main professional and educational association for the geothermal community and public. Primarily deeper geothermal (aka hot rock) used to create electricity, but also includes networked geothermal.